The latest technology developments are boosting the quality control and monitoring of joining processes
Resistance spot welding is the most commonly applied process for joining thin metal sheet in automotive body structures, with a typical family car containing up to 5,000 spot welds. However, one aspect of the spot-welding process that is not tightly controlled is the wear of electrode tips. During welding, mechanical strain and thermal diffusion cause continuous abrasion to the fronts of the welding tips. In turn, the operating lifetime of the welding tip fronts directly affects the quality of fabrication.
Zinc-coated steels are the most popular car body material, but zinc rapidly degrades the electrode to the point where maintenance is required to ensure adequate weld quality. Electrode wear can be repaired by a technique known as 'tip dressing' where a mechanical cutter removes material from the tip face, thus repairing any damage and returning the tip to its optimum geometry.
Today nearly all modern automotive spot-welding lines run production with automated tip dressing, but such systems do not offer a complete solution and problems with electrode tip quality remain the number one cause of stoppages on car production lines. Furthermore, conventional tip dressers remove a large quantity of material, resulting in rapid consumption of expensive copper electrodes.
Among the latest innovations to tackle electrode tip quality is SmartDress, the result of a two-year European research project involving three research institutes and four companies: Fraunhofer IMPS (Germany); The Welding Institute (UK); Teknologian Tutkimuskeskus (Finland); Sinterleghe (Italy); Finishing Aids & Tools (UK); Swantec Software & Engineering (Denmark); and Fisher Smith (UK).
First is an optical sensor able to monitor electrode condition and provide vital data concerning the state of wear and, second, a computerised control system that processes data from the optical sensor to determine the level of electrode cleaning required. The control system can also optimise dressing schedules, adaptively controlling electrode maintenance frequency. The third element of the system is a mechanical dresser designed to minimise copper removal during tip dressing, and the final element is an automated tip changer able to replace worn tips during production without the need for a line stop and manual intervention.
As for the sensor’s function, an assessment of welding tip condition requires views of the front and side. The front view provides information about staining of the tip front, which is needed to decide on the type of whetting procedure; the lateral view shows the diameter of the tip front and the electrode length. The information from both views is used to decide on the necessity of a contour cut, or an exchange if the remaining electrode length is small.
Illumination wavelength
For the various sheet materials used in the welding process, specific types of front staining are observed. Therefore, the illumination wavelength must be adjusted to the particular application. These are chosen to match the absorptive or reflective properties of the respective material which agglomerate at the stained front surface.
For sheet steel, red light is used (absorption of the contaminant), while for aluminium sheets blue light is used (reflection of the contaminant) as a diffuse illumination. The sensor is constructed so that the welding gun locks into place and the electrodes from both sides are measured simultaneously in both views. Depending on the design, three or four cameras are required to do this, one each for the lateral view and one or two for the front view.
Michael Leuckefeld, project manager at Fraunhofer IPMS, says: “Our system monitors both top and lower spot welding electrodes simultaneously from various perspectives. We use diffuse monochrome LED lighting generated by opal glass for this purpose. Light is sent to the electrode via a mirror arrangement and beam splitter, and then back to the photo detector. Lighting and image recording therefore use the same optical elements.”
Weld-seam inspection
In today‘s production environment, automotive manufacturers and their suppliers must adhere to rigorous quality requirements as part of their zero-error strategies. These requirements can only be fulfilled with the assistance of automated weld-seam measurement and inspection technology. Here, machine vision systems are designed to offer reliable and accurate inspection.
The HDR-3D sensor within Vitronic’s VIRO WSI system inspects weld seams on reflective materials such as aluminium, as well as flat laser seams like those found in battery production. Typical applications include the welding of axles, bodywork, exhaust systems and wheels. The system automatically detects and visualises deviations from predefined quality standards. Three-dimensional visualisations of seam sizes, defects (such as pores and holes) and other relevant inspection results combine to enable targeted reworking of parts, while all information about weld-seam quality is documented and archived in an integrated database, with details of the specific seam and part.
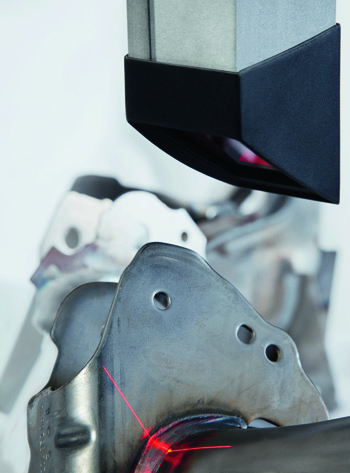
Pareto analyses show any potential for production optimisation. The parameters for quality inspection can be adjusted during the production process and are applied automatically to the next system cycle, thus minimising rework and scrap. Furthermore, 100% inspection ensures that parts are only admitted to downstream manufacturing processes if they are free of defects.
During the software set-up process, all relevant parameters, such as the inspection procedure, thresholds and criteria, are configured in line with the specific production requirements. The company says its VIRO-WSI system is also smaller than the previous-generation unit, while energy consumption has been reduced by 75%.
Retaining the seam theme, Meta Vision Systems has recently introduced its SLS (Smart Laser Sensor) ARC for robotic arc welding applications. It is suitable for seam finding and real-time weld-seam tracking in all of the usual welding processes, including high current GMAW and FCAW.
Steve Thacker, Meta’s general manager, says: “Meta was spun out of Oxford University in 1984 to commercialise a research project that developed a laser vision sensor for use in robotic MIG welding in the automotive industry. The SLS ARC represents the latest development in a 30-year sequence which started with that first Meta sensor.
“The automotive sector represents an important and still growing part of our business, and one where we have had good recent success,” he continues. “We felt it was timely to build on that with a new sensor fine-tuned for aggressive robot welding applications. The ARC is just one part of an overall product development plan aimed at extending the state-of-the-art in robot welding.”
SLS ARC includes automatic image-quality optimisation, combined with new sensor optics and a mechanical design matched to the requirements of robot arc welding. It exploits Ethernet connection from the sensor head directly to the robot controller to give good seam finding and real-time tracking.
Looking at lasers
So, what of laser welding, the automotive sector’s rising star? Well, among recent innovations for the supply chain is Prima Power Laserdyne’s newly developed SmartRamp, which is designed to provide consistent end-point control during laser welding, eliminating areas where potential weld failure can occur. SmartRamp is the latest addition to the Laserdyne S94P control, which is used to operate the Laserdyne 795 and Laserdyne 430BD multi-axis laser welding systems.
In standard laser welding practice, power is often ramped down at the end of the weld after the start point has been overlapped. This method leaves a noticeable depression at the end point, which in many instances is undesirable. For example, in hermetic welds, which are used widely in sealed electronic packages for the automotive sector, this depression can be a leak point. The quality and durability of the hermetic seal in these packages must be high, as failure of the seal can lead to corrosion of the package contents and premature device failure.
By comparison, SmartRamp controls laser parameters in conjunction with beam motion, eliminating the occurrence of a visible weld end point. To validate the technology’s effectiveness, Prima Power Laserdyne carried out extensive metallographic analysis of welds produced using SmartRamp. These consistently showed the absence of porosity or any other welding defects at the end of the weld. In contrast, metallographic tests carried out on standard welding samples confirmed that the end point can be the site of some porosity and inconsistent weld penetration.
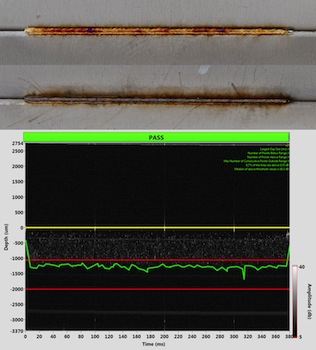
Elsewhere, Laser Depth Dynamics, which has been creating plenty of industry interest with its patented ICI (Inline Coherent Imaging) technology for laser weld depth measurement, has been focusing on developing the solution for laser weld-seam tracking applications. The company says that ICI’s ability to cut through intense optical noise in laser processes and provide high-resolution geometrical data has enabled technology that offers combined auto-focus and seam-tracking capabilities.
Since the ICI system is immune to blinding by the process beam, no additional sensors are required around the processing region. Importantly, the imaging beam shares the same optics as the process beam and, unlike triangulation techniques, requires no additional angular access to the part. As a result, laser weld-seam tracking around corners and through remote optics is possible without the need to rotate the head.
By measuring the distance from the laser head to the work surface before, during and after welding, precise focus and position can be maintained even under unstable conditions. The proximity of the seam-tracking measurement point to the point of interaction is limited only by the melt pool size.
Spot welds that are not fused completely or are undersize are seldom visible to optical inspection, while destructive pull tests are laborious and limited to a few samples. However, using the latest equipment and techniques, ultrasonic spot-weld inspection can quickly and non-destructively provide valuable information about weld quality.
Spot-weld testing requires coupling sound into a cup-shaped weld typically three to six millimetres in diameter and generating multiple back-wall echoes at high frequency. A variety of delay line and captive water column transducers in the frequency range from 10 to 20MHz are available from Olympus for this purpose.
Delay line transducers use a small plastic waveguide to couple sound energy from the transducer element to the test piece, while captive water column transducers incorporate a pliable rubber membrane enclosing a column of water that conforms to the topography of the spot weld to optimise coupling. The delay line diameter and element diameter are usually matched to the nominal nugget diameter to within a few tenths of a millimetre. For captive water column transducers, the element diameter is normally matched to the nominal nugget diameter.
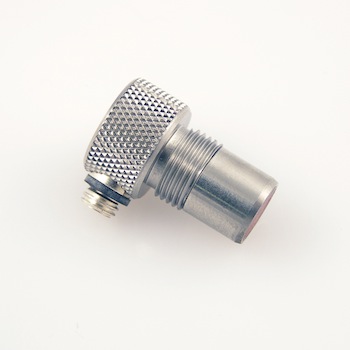
In a good weld, the spacing between echoes will be proportional to the thickness of the weld, and the decay rate (the rate at which successive echoes decrease in amplitude) will be related to the attenuation within the nugget.
There will be a characteristic echo pattern associated with a good weld, such as: if there is no fusion between the two pieces of metal then the successive echoes will be much closer together and larger in amplitude. In the case of an undersized weld, a portion of the sound beam will reflect from the total thickness of the two metal sheets, while a portion reflects from a single thickness. This creates a pattern in which small peaks representing single-sheet thickness appear between the larger and more widely separated peaks representing the full weld thickness.